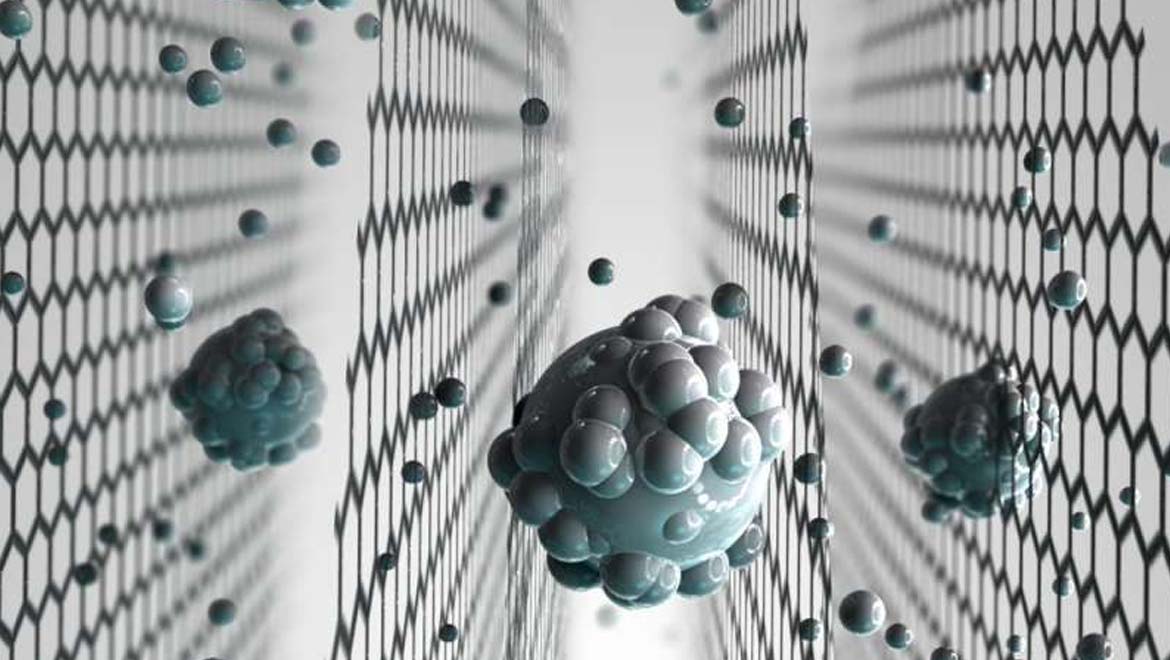
The Role of Graphene in Water Desalination
Extensive environmental pollution caused by worldwide industrialization and population growth has led to a water shortage. This problem lowers the quality of human life and wastes a large amount of money worldwide each year due to the related consequences. One main solution for this challenge is water purification. State-of-the-art water purification necessitates the implementation of novel materials and technologies that are cost and energy efficient. In this regard, graphene nanomaterials, with their unique physicochemical properties, are an optimum choice. These materials offer extraordinarily high surface area, mechanical durability, atomic thickness, nanosized pores and reactivity toward polar and non-polar water pollutants. These characteristics impart high selectivity and water permeability, and thus provide excellent water purification efficiency. This review introduces the potential of graphene membranes for water desalination. Although literature reviews have mostly concerned graphene’s capability for the adsorption and photocatalysis of water pollutants, updated knowledge related to its sieving properties is quite limited.Role of Graphene Role of Graphene
So far, seawater desalination has been mainly performed via multistage flash distillation and reverse osmosis (RO).6 Mostly in the arid Persian Gulf countries, desalination plants perform based on heating and then condensing seawater. This kind of desalination plant consumes large amounts of thermal and electric energy, thus emitting greenhouse gases extensively.7 In addition to this non-economical and non-ecofriendly version of desalination plants, the main type of desalination plants constructed in the past two decades, as well as future planned ones, are based on RO technology.
In the RO process, water is forced through a semi-permeable membrane by an external positive hydrostatic pressure. Accordingly, a larger volume of water passes through the membrane compared with the volume of dissolved salts or organic molecules.2 Thanks to economical and technical efficiencies regarding the desalination of seawater on a large scale, over 50% of the entire desalination capacity is supplied by RO systems.1, 2 RO is indeed the most energy-efficient technology for seawater desalination and is the benchmark for the comparison of any new desalination technology. The level of energy consumption has even declined in the past 40 years,8 owing to technological improvements in the development of membranes with higher permeability and the installation of energy recovery devices and highly efficient pumps. However, this technology still suffers from low desalination capacity and high capital costs, hampering its broad application in most developing markets.2 Thus, research is in progress to overcome such limitations and to create more efficient membranes.
Graphene can be regarded as an ‘ultimate’ RO membrane, because it is stronger, thinner and more chemically resistant than the polyamide active layers in thin-film composite RO membranes. In fact, water flux across a membrane is inversely proportional to its thickness. Thus, the atomic thinness of graphene (d≈0.34 nm) can lead to larger water permeability than the polyamide active layer in thin-film composite membranes (d≈100 nm). In addition, graphene shows better tolerance to chlorine than polyamide, that is, an important advantage in hindering membrane fouling without degradation.12 Graphene as graphene oxide (GO) shows antimicrobial properties, thus lowering membrane biofouling, that is, improving the membrane lifetime and energy consumption of the water purification processes.14, 15 Moreover, graphene can readily be processed into a membrane for application as RO and nanofiltration (NF) (a low-cost and highly efficient separation technique between ultrafiltration and RO) desalination membranes. For such reasons, extensive research is currently being carried out to realize its potential as a next-generation desalination membrane.12
One of the most serious problems adversely affecting people around the world is insufficient access to clean and potable water and sanitation. This problem will expand in the coming decades as water scarcity begins to occur globally. To address this challenge, extensive research must be conducted to identify advanced novel methods of water purification at lower cost and with less energy. In addition, such technologies must be independent from the use of chemicals and must not impact the environment.
Graphene nanomaterials offer novel solutions for water purification and facilitate the development of advanced water purification membranes, especially for water desalination. Owing to its atomic thickness, assuring high fluid permeability and thus energy/cost efficiency, extraordinary mechanical stability and potential for size-selective transport, graphene is an ideal candidate for future membranes. Graphene-based membranes possess several fascinating advantages over conventional membranes. First, their raw material is graphite, that is, an inexpensive material that affords low membrane fabrication costs. Second, the fabrication procedure of graphene membranes, for example, the fabrication of GO membranes based on GO nanosheets, is quite simple and scalable and enables the technical readiness for scaling up membrane production. This kind of membrane can be further improved by engineering the spacing between the GO layers through the inclusion of different-sized cross-linkers. In addition, the membrane charge and thus the charge-based selectivity can be modified by functionalizing GO with various functional groups.
However, to close the gap between research at the lab scale and practice at the industrial scale, there are still some challenges that must be overcome. First, the selectivity of graphene multilayer membranes is mainly limited to large organic molecules and hydrated ions. Thus, the membranes can perform solely as ultrafiltration or NF membranes. To extend their applicability to desalination, that is, to obtain high salt rejection efficiency, the nanochannel size must be tailored in the subnanometer range. Moreover, if seawater desalination is the target application, realistic salt concentrations and thus solvated ion concentrations should be considered. At the moment, the studied membranes are able to offer the efficient removal of low amounts of salt of a few tens of mM (versus the average value of 0.6 M for seawater), which is not applicable for large industrial scales. Accordingly, the design and modification of graphene-based membranes should be properly done and, in fact, revolutionized. Considering the high surface area/mass ratio of graphene nanomaterials, this goal could be met by advanced design strategies of, for example, membrane modules exposing large surface areas or by the insertion of negatively charged functional groups, maximizing the electrostatic repulsion with ions. As mentioned earlier, at the moment, the developed graphene membranes can be employed solely as NF membranes, not to reduce seawater salinity to drinking water standards, but to treat mildly brackish feed water. However, by coupling with RO, the membranes can be used in seawater desalination. In addition, future research is required to thoroughly comprehend the transport mechanism of water and solutes in such membranes. In parallel, other potential separation mechanisms (for example, charge and adsorption effects) in addition to sieving must be explored. In this regard, it is necessary to create multifunctional graphene membranes with exceptional antifouling, adsorptive, antimicrobial, and photocatalytic properties. Moreover, for application in environmentally benign and green applications, the long-term stability of graphene membranes in aqueous systems should be improved. This feature can be tracked by ecotoxicological characterizations to guarantee the effective application of graphene membranes and minimize the risks related to possible relevant adverse health and environmental effects. In addition, the mechanical strength and durability of graphene membranes is still a challenge that restricts their practical application. In this regard, employing potential supports for graphene membranes or incorporating them into a host matrix could be a solution. Last but not least, the large-scale, controlled production of graphene membranes for industrialization is still a challenge and needs further study to be realized.